TREX’s Small Bodies theme considers analysis of data related to main belt and near-Earth asteroids. The studies examine various physical and chemical properties of these objects important to determining their potential to provide resources for space exploration. Potential resources translate into ‘useable materials’, which requires 1) understanding the composition of materials on these asteroids, 2) finding out where these materials reside on and within these asteroids, and 3) determining whether they are accessible. Understanding these bodies is also essential to understanding the origins and evolution of our solar system. There are a variety of studies that, at first may seem disconnected, but when examined closely help us form a complete picture of these small objects.
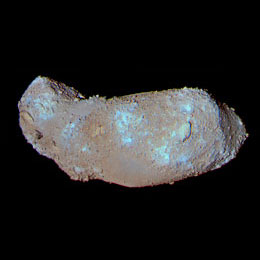
Small bodies are scattered throughout our solar system, and we will study them through both remote sensing and laboratory science.
How do we learn the composition of materials on an asteroid?
There are many types of measurements that can be used to address this seemingly simple question. These include: 1) measuring the spectrum of reflected light from the surface (this tells us about the top micrometers to millimeters of the surface, and tells us mineral composition), 2) measuring gamma rays and neutrons produced by cosmic ray interactions with subsurface materials (this tells us about the elemental composition of the outermost meter of the surface). The data can be acquired remotely from an orbiting spacecraft or in place from a rover or lander. Together, elemental and mineral data provide clues to the origin, formation, and evolution of a small body. They provide the first insights into potential resources present on an asteroid or comet of interest.
But there’s a twist: the space environment in which asteroids, especially near-Earth orbiting objects (NEOs) reside, is hostile and the surface mineralogy can be altered by a variety of physical and chemical processes. The dominant processes associated with the space environment are: 1) micrometeorite impacts, 2) radiation bombardment from the solar wind, and 3) thermal effects due to changing distances from our heat source, the Sun. We can understand these physical and chemical alterations using laboratory measurements and by modelling the dynamical evolution of these objects from the main-belt to near-Earth orbit.
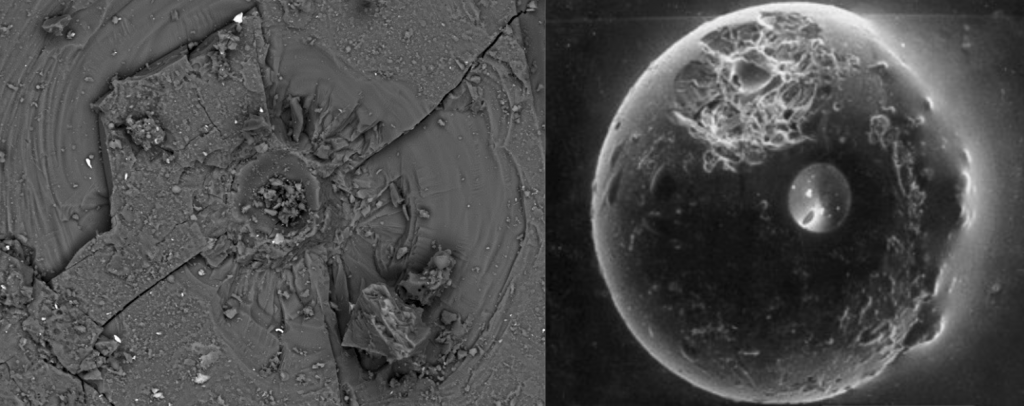
Micrometeorites are tiny, micron-sized particles, which when they impact a surface create tiny impacts on the grains of the material lying on the surface. These impacts create craters (roughing up the surface), impact melt and ejecta (covering the grain with glass), and they also create submicroscopic materials, such as submicroscopic metallic iron (smFe0), which is observed in lunar samples, and submicroscopic iron sulfide (smFeS), which is found in the returned Itokawa asteroid samples. Radiation from the Sun also creates these submicroscopic materials by implanting hydrogen into an iron- and oxygen-bearing mineral, creating water or hydroxyl (H2O/OH) and metallic iron. The presence of these particles affects the reflectance properties of the surface, which is seen in the spectral observations.
Spectral models designed for the Moon incorporate submicroscopic Fe0 (smFe0) for the space-weathering products that darkens and reddens the spectra and diminishes absorption features due to the presence of SmFe0 in mineral rims. However, for Fe-poor and/or C-rich bodies, submicroscopic carbon (smC) may be the main space-weathering product that alters the spectral signature. We have adapted models designed for the Moon to include various forms of carbon (amorphous carbon, graphite) in place of Fe-metal. These models predict how the spectrum would be altered by the presence of smC in mineral rims.
But modeling is not enough. We are also going into the laboratory to test and validate these models. At the Johnson Space Center (JSC) we are using their vertical gun impact facilities to mimic micrometeoritic impacts. We are measuring the spectral effects of these impacts. These experiments have begun using phyllosilicates, water-rich minerals present on many asteroids (commonly called clays). We are preparing to also run radiation experiments to mimic how the Sun’s “wind” affects minerals present on asteroid surfaces. These experiments will be run over the course of the next couple years.
The quest for resources begins with understanding where the resources are most likely to reside. Asteroids that have orbits near to Earth’s orbit are easiest to travel to. How likely are they to have the resources we need for exploration? One way to answer that question is to examine how asteroids in the main belt, that are known to have water on their surface, can migrate to become objects whose orbit crosses that of Earth’s. Examining the evolution of their orbit, how close and how often they approach the Sun, can provide information on how well they retain those resources over their lifetime. We are currently modeling main-belt Themis family asteroids to understand their migration and retention of water once in the near Earth orbit.
How do we understand ‘where’ materials of interest can be found? Which leads to ‘are they accessible?’
Once we identify the composition of materials available, we next ask ‘can we get to them easily, or with what kind of effort?’ Is the material we are interested in present on the surface in big chunks or as fine-grained powder? Is the material we are interested in present in layers, or mixed in with other materials, or present as inclusions in grains or rocks? One group of studies that can help address these types of questions, when coupled with the UV-IR and nuclear spectroscopy, are photometric studies. Photometry examines how the reflectance of light from the surface changes as the illumination angles and observation angles change. Models exist that correlate these changes with grain size, porosity, and grain structure.
Nuclear spectroscopy probes deeper into the surface than optical methods, such as photometry and UV-IR spectroscopy, and provides information about the elements that make up the minerals found in the surface. Neutron spectroscopy is very sensitive to elements found in ices (an important resource needed for exploration). In many locations in the solar system, water ice is unstable on the surface, but can survive at depth, hidden from the optical instruments. The rate at which neutrons escape the surface is strongly affected by the presence of hydrogen, found in water, organics, and hydrated mineral species. As a result, the presence of hydrogen-rich materials in the subsurface, such as water, can be inferred from neutron spectroscopy. For example, the NASA Dawn mission’s Gamma Ray and Neutron Detector (GRaND) detected the presence of subsurface water ice on the dwarf planet Ceres, the largest body in the main asteroid belt. Water ice is likely hidden beneath the surface of many small bodies in the main asteroid belt, providing a potential resource that could be used by future explorers.
Of course, mineral and elemental data together are required to fully understand the chemistry of small bodies. For example, mineralogy from optical spectroscopy can provide detailed information about the types of hydrous minerals on the surface. This information when combined with nuclear spectroscopy can be used to determine the concentration and layering of water ice in the near subsurface. . As a result, a primary goal of our theme is to understand through modeling how mineral and chemical data can best be combined to characterize surface compositions.
Finally, spin-off applications of technology are commonplace and planetary science is no exception. GRaND derives its heritage from instruments flown on previous missions, including Apollo, Lunar Prospector, and Mars Odyssey. Similar instrumentation and methods are used to search for mineral resources deep beneath the surface of the Earth. An example spin-off of GRaND technology is the use of passive gamma ray measurements of natural radioactive elements (K, Th, and U) to support field studies in TREX Theme 4. Another is in-cave measurements of atmospheric muons to determine the physical properties of the surrounding rock. Nuclear instrumentation can provide data to constrain physical properties of the rock formation, providing new information that can be used in a variety of cave science studies.